Investigation of Spark Plasma Sintering Temperature on Microstructure and Thermoelectric Properties of p-type Bi-Sb-Te alloys
Article information
Abstract
In this work, p-type Bi−Sb−Te alloys powders are prepared using gas atomization, a mass production powder preparation method involving rapid solidification. To study the effect of the sintering temperature on the microstructure and thermoelectric properties, gas-atomized powders are consolidated at different temperatures (623, 703, and 743 K) using spark plasma sintering. The crystal structures of the gas-atomized powders and sintered bulks are identified using an X-ray diffraction technique. Texture analysis by electron backscatter diffraction reveals that the grains are randomly oriented in the entire matrix, and no preferred orientation in any unique direction is observed. The hardness values decrease with increasing sintering temperature owing to a decrease in grain size. The conductivity increases gradually with increasing sintering temperature, whereas the Seebeck coefficient decreases owing to increases in the carrier mobility with grain size. The lowest thermal conductivity is obtained for the bulk sintered at a low temperature (603 K), mainly because of its fine-grained microstructure. A peak ZT of 1.06 is achieved for the sample sintered at 703 K owing to its moderate electrical conductivity and sustainable thermal conductivity.
1. Introduction
Thermoelectric conversion is an emerging technology which has a capable of converting waste heat energy to electric energy (power generation), and electricity into cooling (refrigeration). The conversion efficiency is defined by a dimensionless constant called figure of merit, ZT = (α2σ/κ)T, where α is the Seebeck coefficient, σ is the electrical conductivity, κ is the thermal conductivity, and T is the absolute temperature respectively [1, 2]. In the past decades, there were several thermoelectric (TE) materials have been introduced to achieve high ZT. Among them, bismuth-antimony-tellurium (Bi-Sb-Te) based alloys exhibit best thermoelectric properties at room temperature (300K) [3-5]. Since the discovery of thermoelectricity, the convensional single crystal processing methods including zone melting [6] and Bridgman [7] methods are promising and popular for manufacturing TE materials. Despite their excellent thermoelectric properties, they have numerous disadvantages such as low mechanical properties due to ease of cleavage along their basal planes, and has a high-energy consumption & low productivity [8]. To overcome these disadvantages, various researchers have been progressing to improve the characteristics of thermoelectric and mechanical properties by preparing a powder using the powder metallurgical processes followed by a various vacuum sintering technologies [9-11]. Gas atomization is an effective largescale method of producing thermoelectric powders by a high pressure inert gas [12]. Since the solidification of the alloy is performed for a short time, a homogeneous and uniform microstructure with a uniform chemical composition and particle size can be produced. The consolidation of thermoelectric powders is a crucial part in which the microstructural changes with densification could significantly affect their transport and thermoelec- tric properties. There are listed few of well sintering methods such as hot pressing (HP) [13], hot extrusion (HE) [11], spark plasma sintering (SPS) [12, 14], and hot deformation (HD) [15]. Among them, the spark plasma sintering is an excellent method of producing a sintered body having a high density by performing sintering at short duration. The grain growth could eminently control during the SPS since it is rapid sintering process, which can effectively improve mechanical properties due to grain boundary hardening. In addition, the anisotropy in thermoelectric materials can neglect in SPS process in which the sintering body having uniform physical and electrical properties [12]. It is known that the microstructure plays crucial role to improve thermoelectric properties, thus it is necessary to control the microstructure. Recently Wan-Ting et al argued that the SPS sintering temperature can eminently affects the Sb2-xInxTe based thermoelectric properties, in which the highest σ/K is essential to achieve higher ZT values at moderate sintering conditions [16]. Thus, optimization of sintering temperature is one of the primary thing in consolidation process to achieve adequate microstructure with improved thermoelectric properties.
In this study, p-type Bi-Sb-Te thermoelectric powders with excess Te were prepared by gas atomization. Subsequently, the gas atomization powders are consolidated by SPS process at a different sintering temperatures. The microstructures, and thermoelectric properties are systematically investigated as a function of sintering temperature.
2. Experimental procedure
To make the stoichiometric Bi0.5Sb1.5Te3 excess Te composition, we have taken Bi, Sb and Te granules with high purity (99.999%), purchased from Alfa acer, and thermoelectric powders were prepared by the gas atomization method [12]. The gas atomized powders were mechanically sieved under 200 μm for the present work. The selected powders consolidated using spark plasma sintering with a graphite mold with an inner diameter of 25 mm. The powder was sintered at different sintering temperatures such as 663K, 703K, and 743K with maintaining co-axial pressure of 50 MPa for 10 min.
The density of the sintered body was measured using Archimedes' method. Micro Vickers hardness tester was used for hardness. The phase of the sintered bulks were analyzed with X-ray diffraction (MiniFlex 600, Rigaku). The fracture surface was characterized by scanning electron microscopy (MIRA LMH, TESCAN). Moreover, electron backscatter diffraction (JSM-7000F, JEOL) was used to observe the grain distribution and orientation of the grains in sintered bulk. The electrical conductivity and seebeck coefficient of the thermoelectric materials were measured by thermopower measurement system (TEP-1000, Seepel) at 300~400K temperature range. Power factor was calculated using measured electrical conductivity and seebeck coefficient. The carrier concentration and mobility values were measured using a Hall effect (HMS-3000, Ecopia). The thermal conductivity was measured by the thermal diffusivity (LFA-457, NETZSCH) and the specific heat. Figure of merit (ZT) was calculated using electric conductivity, the seebeck coefficient and the thermal conductivity.
3. Results and Discussion
The phase of the initial gas atomized powders, and sintered samples (at different temperatures) were characterized by X- ray diffraction (XRD), and pattern were shown in Fig. 1. The diffraction pattern could be indexed to the Bi0.5Sb1.5 Te3 phase with a rhombohedral structure (JCPDS #49-1713), indicating that all samples were in single phase, and no impurity phases were observed. Besides, the orientation factor was calculated as 0.015, 0.008, and 0.006 for samples sintered at 663K, 703K, and 743K respectively. This results indicating that the orientation was very small (no preferred orientation in any special direction), and can be negligible. The relative densities were measured and obtained over 99.5% of its relative density. As prescribed earlier that the spark plasma sintering technique was a rapid consolidation with full densification technique. The Vickers hardness was measured using hardness tester and shown in Table 1. The maximum hardness of 72.7Hv was obtained for sample sintered at 663K. Since grain growth behavior, the hardness values were decreased with increase in sintering temperature. However, the obtained hardness values were higher than those single crystal grown methods [17].
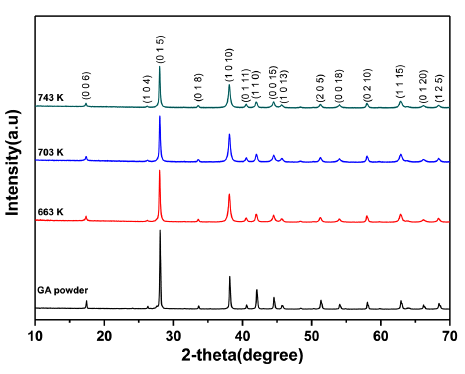
X-ray diffraction patterns of Bi0.5Sb1.5Te3 thermoelectric powder by gas atomization and SPS sintered bulks at different sintering temperatures.

Relative density and Vickers hardness of Bi0.5Sb1.5Te3 samples sintered at 663K, 703, and 743K respectively
In order to analyze the bulk microstructure, grain distribution, and texture in bulks; the bulk fracture surfaces and EBSD texture analysis were treated. Fig. 2 shows the scanning electron micrographs of fractured surfaces of a p-type Bi0.5Sb1.5Te3 bulks sintered at (a) 663K, (b)703K, and (c) 743K respectively. It was observed that the grains are randomly distributed on the matrix, with combination of submicron-order, and micron order range. This kind of bi-modal distribution of grains were from the fine and large sized gas atomized powders (few micrometers range to below 200 μm), as reported in our earlier reports [12]. The bimodal microstructures can favor for increase in Seebeck coefficient, and reduction in thermal conductivity, extensively due to the scattering of carriers taking places at grain boundaries in complex bimodal microstructures. In addition, many of grains were exhibited laminar structured along the c-axis regardless of sintering temperatures, which was resulted from the inherent crystal behavior of Bi2Te3-based alloys [8]. Further, texture analysis was analyzed through EBSD, and shown in Fig. 3. As prescribed in the earlier, the grain size was intentionally increased as increasing sintering temperatures from 663K to 743K as shown in Fig. 3(a)-(c). Each colored region/domain was representing an individual grain, and texture orientation in unique direction. It revealed that the texture was randomly orientated in entire matrix, and no such kind of preferred orientation in any unique direction was observed. This was a good agreement with the XRD pattern analysis in which no basal plane intensities were orientated. The increase in grain size could severely affected the transport properties in thermoelectric materials. It was known that the large grains can induce the high carriers transport from one to another, as compared with fine grains. Such kind of carrier transportation behavior can increase the carrier mobility in matrix, which benefits to increases in electrical conductivity.
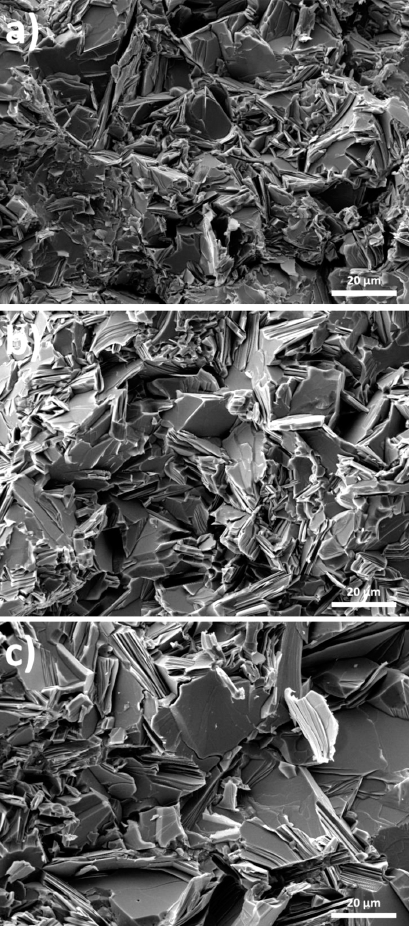
SEM Fractured surfaces of Bi0.5Sb1.5Te3 bulks fabricated by SPS at a) 663K, b) 703K, and c) 743K temperatures. The fracture surface represents bi-model microstructure of bulk samples regardless of sintering temperatures.
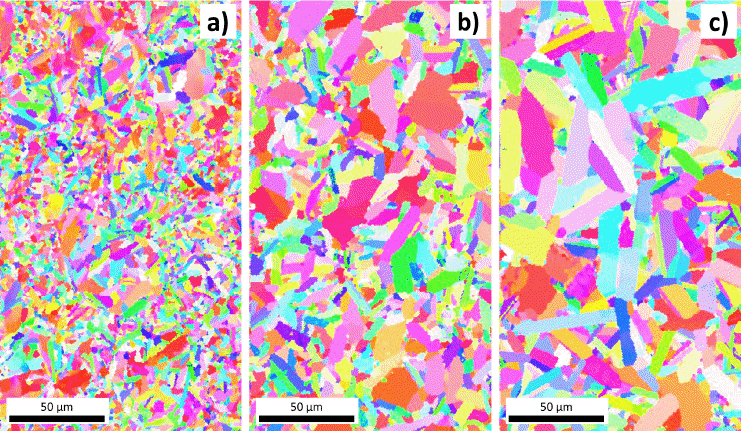
Texture analysis of Bi0.5Sb1.5Te3 bulks fabricated by SPS at a) 663K, b) 703K, and c) 743K temperatures. The texture analysis indicating no preferred orientation in any direction.
The temperature dependence of the thermoelectric properties of p-type Bi0.5Sb1.5Te3 samples with different temperatures were shown in Fig. 4. All measurements were carried out along the direction perpendicular to the SPS pressing direction. The electrical conductivity (shown in Fig. 4(a)) decreased with temperature for all samples, exhibiting a degenerate semiconductor behavior. The electrical conductivity increased gradually with increase in sintering temperature and can be attributed to the increases in carrier mobility (μ), as shown in Fig. 4(b). The peak electrical conductivity of 1016 Ω-1 cm-1 was obtained for the SPS-743K sample at RT. In general, the electrical conductivity (σ) can be expressed as [12]
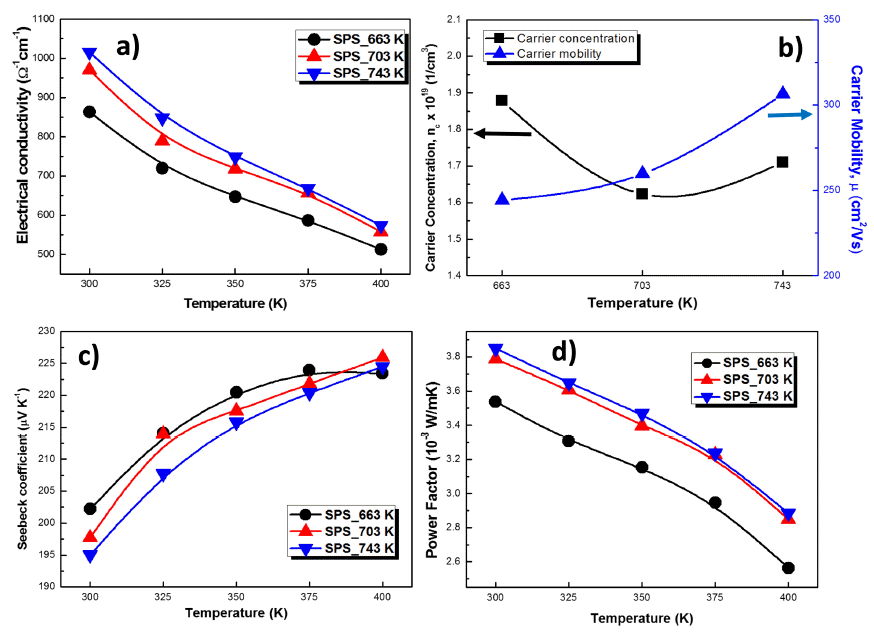
Temperature dependence of (a) electrical conductivity, (b) carrier concentration, and mobility, (c) Seebeck coefficient, and (d) Power Factor of Bi0.5Sb1.5Te3 bulks fabricated by SPS at different temperatures.
where n is the carrier concentration, and e is the carrier charge. The measured n, and μ were shown in Fig. 4(b). The carrier mobility increased with increasing sintering temperature from 663K to 743K, which was attributed to the increase in grain size. Besides, the carrier concentration was slightly decreased with sintering temperature. However, the magnitude of the increase in mobility was high, which was caused to increase in electrical conductivity with sintering temperatures.
The temperature dependent Seebeck coefficients (α) of Bi0.5Sb1.5Te3 samples with different temperatures were shown in Fig. 4 (c). All sintered samples show positive Seebeck coefficient values indicating a p-type semiconducting behavior. The Seebeck coefficient values of 202, 197, and 195 μV/K obtained for the samples sintered at 663K, 703K, and 743 K at RT respectively. It was noticed that the α values decreased with increase in sintering temperature mainly due to the increase in carrier mobility.
It is known that the Seebeck coefficient (α) of p-type Bi0.5Sb1.5Te3 materials were severely influenced by the carrier mobility, can be represented as follows [4, 12]
where kB is the Boltzmann’s constant, h is the Plank’s constant, τ is the relaxation time [(eτ/μ) = m*]. The increase in carrier mobility should be severely affected the Seebeck coefficient, as from the expression (2), thus finally decreased its values with increase in sintering temperature. The power factor, PF can be calculated from the square of the Seebeck coefficient with electrical conductivity was shown in Fig. 4(d). The power factor values decreased with increase in measurement temperature as like electrical conductivity. The peak PF of 3.85 W/mK2 was obtained at RT for SPS-743K sample, and decreased its value with decrease in sintering temperatures. The increase in electrical conductivity could be more contributed to the increase in power factor for the samples with sintering temperatures.
Fig. 5 shows the thermal transport properties of p-type Bi0.5Sb1.5Te3 samples at different temperatures. The total thermal conductivity κtot is the sum of electronic component κe, and the lattice component κL. The electronic thermal conductivity can be calculated by the Widemann- Franz Law; κe = σLT, where L is the Lorentz number, σ is the electrical conductivity, T is the absolute temperature, respectively [18]. The total thermal conductivity was shown in Fig. 5(a), and noticed that κtot was minimum for low sintering temperatures, and increased as increase in sintering temperatures. In order to calculate that electronic component of thermal conductivity, Lorentz number should be determined. The Lorentz number is estimated from the single parabolic model, and plotted in Fig. 5(b). The estimated Lorentz values were lower than the degenerate semiconductor materials (L=2.45×10-8 WΩK-2) [19]. The electronic components of thermal conductivity (κe) can be calculated by using estimated Lorentz numbers, and drawn in Fig. 5(c). The κe values decreased with increase in sintering temperature, which is proportional to the electrical conductivity. Further, the lattice thermal conductivity, (κL) (shown in Fig. 5(d)) was low at low sintering temperatures, suggesting that fine grains were inhibit the phonon propagation in matrix. Finally, the obtained low κtot are mainly attributed to the fine grain microstructure at low sintering conditions, in which the carriers/ phonons can eminently blocked at fine grain boundaries [10]. The reduction thermal conductivity could benefit for the enhancement of figure of merit, discussed in the following section.
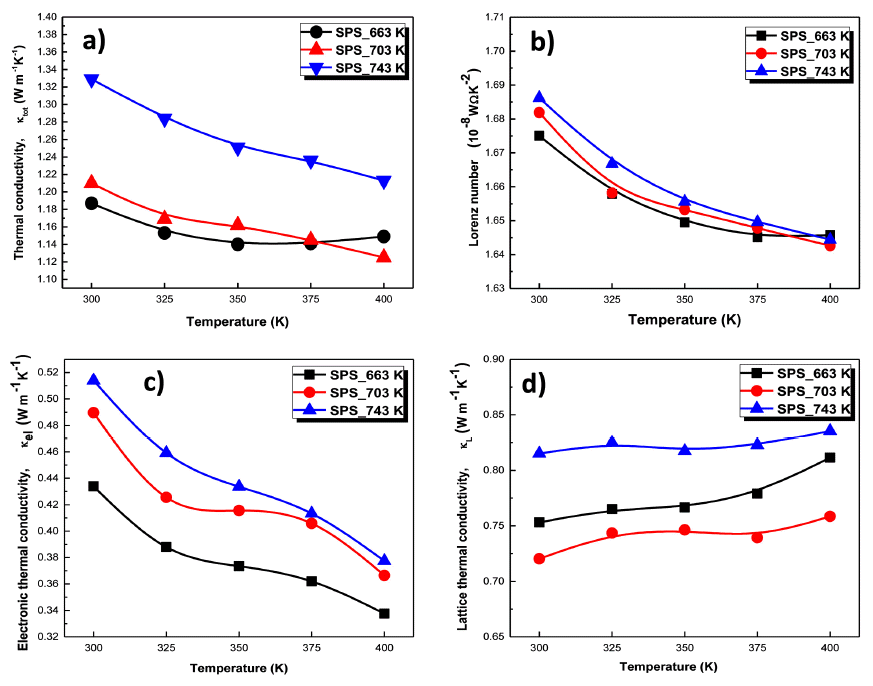
Temperature dependence of (a) Total thermal conductivity, (b) Lorentz number, (c) electronic thermal conductivity and (d) Lattice thermal conductivity of Bi0.5Sb1.5Te3 bulks fabricated by SPS at different temperatures.
The temperature dependence of the figure of merit, ZT was shown in Fig. 6. It was evident that the sample sintered at 703K shows high ZT values than other samples. The peak ZT of 1.06 was achieved for the SPS-703K sample at 375K. It should be noted that the SPS-703K sample exhibit moderate electrical conductivity with sustainable thermal conductivity values, this could cause to increase in Figure of merit. The improved large-scale production of p-type Bi0.5Sb1.5Te3 samples by gas atomization and SPS can beneficial for commercialization of thermoelectric applications.
4. Conclusion
P-type Bi-Sb-Te alloys powders were successfully produced by gas atomization process, and consequently consolidated with spark plasma sintering (SPS) at 603K, 703K, and 743K. The phase structure of both powders and sintered bulks were analyzed by X-ray diffraction. The severe grain growth behavior was observed with increase in SPS sintering temperature, which would mainly influence the mechanical, and thermoelectric transport properties. The hardness was gradually decreased with sintering temperature, which was mainly attributed to the grain growth behavior. The electron back scattering diffraction analysis revealed that the grains were randomly orientated in entire matrix, and no grains were preferably orientation in any unique direction. The temperature dependence of electrical conductivity increased with sintering temperature, while the Seebeck coefficient decreased slightly due to the increases in carrier mobility with grain size. The maximum ZT of 1.06 was achieved for the sample sintered at 703K due to exhibiting their favorable electrical conductivity and thermal conductivity values.
Acknowledgement
This work was supported by the Korea Institute of Energy Technology Evaluation and Planning (KETEP) granted financial resource from the Ministry of Trade, Industry & Energy, Republic of Korea (No.20152020001210).